The CEMB Principal Investigators represent a diverse array of institutions and scientific backgrounds, all contributing to research that aims to answer pressing questions in the field of mechanobiology.
Charles Anderson, PhD
Charles Anderson, Ph.D., is an Associate Professor of Biology at Penn State University.
Our lab studies cell wall dynamics in plants. Plant cell walls contain networks of carbohydrates and proteins that interact with each other and change in composition and organization during cell growth. We seek to measure changes in cell wall architecture during growth, to understand how interactions between cell wall components influence cell wall structure and remodeling, and to identify and characterize new genes that influence cell wall dynamics. This research will inform efforts to use plant cell walls, which are an abundant and renewable resource, to provide sustainable food, materials, and energy.
Treena Arinzeh, PhD
Treena Livingston Arinzeh, PhD is a Professor of Biomedical Engineering at Columbia University. Dr. Arinzeh received her B.S. from Rutgers University in Mechanical Engineering, her M.S.E. in Biomedical Engineering from Johns Hopkins University, and her Ph.D. in Bioengineering from the University of Pennsylvania. She worked for several years as a project manager at a stem cell technology company, Osiris Therapeutics, Inc. Before moving to Columbia University, Dr. Arinzeh was faculty at NJIT as one of the founding faculty members of the department of Biomedical Engineering and served as interim chairperson and graduate director. Her most notable or cited work to date has been in the use of allogeneic mesenchymal stem cells (MSCs) with bioactive ceramics to induce bone formation in a large bone defect without the use of immunosuppressive therapy. This study served as the basis for FDA approval to pursue clinical trials using allogeneic MSCs for various applications.
Joel Boerckel
2018 SEED project summary: Cells are able to respond to a variety of mechanical stimuli, from fluid shear blood vessel cells, to the changing mechanical properties of the environment in which a cancer cell resides. Cells interpret these mechanical stimuli through a process called “mechanosensation” and translate them into the biochemical language of the cell through “mechanotransduction.” It is often assumed that the progression of mechanosensation to mechanotransduction is a one-way-street, but there is evidence that the biochemical products of mechanotransduction can also regulate how the cells performs mechanosensation. The goal of this project is to understand how these mechanotransductive feedback loops work.
Siobhan Braybrook
Siobhan A. Braybrook, Ph.D., is an Assistant Professor of Molecular, Cell, and Developmental Biology at UCLA.
The Braybrook Team’s research interests center on how multicellular organisms with cell walls grow. Cell walls represent a physical control mechanism for cell proliferation and expansion and they are a biological material with mechanical properties relevant for growth. The Team’s projects range from understanding the molecular mechanisms by which cell wall mechanics are regulated, the physiological aspects of walled-cell growth, and the multi-scale impacts of cell growth patterns or organismal growth and success. The Team also develops methods to measure and interpret cell wall mechanical properties in vivo and using in vitro material mimics.
Keywords: cell wall, biomaterials, plant, algae, growth physiology, multicellular and multiscale growth, materials testing, mechanobiology
Jason Burdick
Jason Burdick, Ph.D., is Bowman Endowed Professor, BioFrontiers Institute and Department of Chemical and Biological Engineering, at University of Colorado Boulder.
His research interests include developing polymeric biomaterials that can be used for tissue engineering, drug delivery, and to investigate cell-matrix interactions. The platform technology involves the engineering of crosslinkable polymers where biophysical and biochemical properties of formed networks can be tailored through material design. These engineered matrices can be used to address questions related to mechanobiology, such as how dynamic changes in material mechanics regulate temporal changes in signaling and differentiation and how material structure alters mechanosensing.
Keywords: polymeric biomaterials; cell-matrix interactions; biomaterials; cell mechanosensing; tissue engineering; bioengineered therapeutics; devices and drug delivery; mechanobiology
Anders Carlsson
Anders Carlsson, Ph.D., is Professor of Physics at Washington University in St. Louis. He is a Co-Lead of the CEMB Working Group 1.
The Carlsson Research Group is focused on modeling the polymerization processes underlying bending of cell membranes. Processes such as membrane protrusion, endocytosis, cell division, and cell migration often involve polymerization and depolymerization of the protein actin. This protein forms long, fairly stiff filaments, whose growth can supply the mechanical force necessary for membrane bending. The filaments are often found in branched or cross-linked networks. The modeling work treats these phenomena with methods including Brownian-dynamics and stochastic-growth simulations, and analytic theory. The main goals of the modeling are to explain how actin generates large pulling forces, to pin down the negative-feedback interactions that are present between actin and its nucleators, and to establish how type-I myosins can aid force generation. This work is being performed in collaboration with Professor John Cooper at the Washington University School of Medicine.
Keywords: membrane bending; actin; Brownian-dynamics; stochastic-growth simulation; analytic theory; mechanobiology
Working Group(s): Working Group 1: How do cells sense their mechanical environment?
Christopher Chen
Christopher Chen, M.D., Ph.D., is Professor of Biomedical Engineering and Materials Science Engineering and Primary Investigator of the Tissue Microfabrication Lab at Boston University. Chen is Associate Director of Research for the Center for Engineering MechanoBiology.
Prof. Chen’s research interests include the application of microfabrication and nanotechnology to cell and tissue engineering, and regenerative medicine. His lab has developed approaches to control the nanoscale adhesive interactions between cells and their surrounding scaffolds, and uses them to control cell function. Prof. Chen is particularly engaged in understanding how to engineer stem cell function and tissue vascularization, and the relationship between tissue architecture and tissue function.
Keywords: cell interaction with environment and subsequent effect on cell function; cell signalling; angiogenesis; stem cell biology; cell adhesion; cell mechanics; micro- and nanofabrication tools; molecular tools; mechanobiology
Working Group(s): Working Group 1: How do cells sense their mechanical environment?; Working Group 2: How do cells adapt to and change their mechanical environment?; Working Group 3: How do cells remember their mechanical environment?; Working Group 4: Crosscutting and Emerging Technologies
Daniel Cosgrove
Cell expansion plays a crucial role in shaping the form and size of plants. My research focuses on the cellular and molecular mechanisms of cell expansion. By use of biophysical, biochemical and molecular techniques, in combination with whole-plant measurements, we are trying to determine (a) which processes limits growth under normal and stressed conditions and (b) how plants regulate their growth rates.
Derrick Dean
Derrick Dean, Ph.D., is Professor of Biomedical Engineering at Alabama State University and Primary Investigator of ASU’s Integrated Bio-Engineering & Advanced Materials (I-BEAM) Center. Dean is the Director of Diversity for the Center for Engineering MechanoBiology.
Dean has conducted leading research in the development of micro- and nanostructured polymer composites, applied in a variety of fields ranging from tissue engineering, drug delivery, aerospace, and the automotive industry. Dean’s research lab specializes in using nano- and microfabrication techniques to synthesize multiscale, multifunctional materials for biological and non-biological applications.
Keywords: micro- and nanostructured polymer composites; scaffolding; tissue engineering; drug delivery; mechanobiology
Working Group(s): Working Group 2: How do cells adapt to and change their mechanical environment?; Working Group 3: How do cells remember their mechanical environment?; Working Group 4: Crosscutting and Emerging Technologies
Keywords: nanostructured materials; spintronics; fabrication and modeling of nanostructured materials; time-resolved imaging of spin dynamics; electrochemical deposition; x-ray magnetic circular dichroism studies; nanoscience; mechanobiology
Working Group(s): Working Group 2: How do cells adapt to and change their mechanical environment?
Dennis Discher
Dennis Discher, Ph.D., is the Robert D. Bent Professor and Professor of Chemical and Biomolecular Engineering, Bioengineering, and Mechanical Engineering and Applied Mechanics at the University of Pennsylvania.
Dennis’ research efforts use synthetic polymers and various micro/nano- tools to understand fundamentals in mechanobiology and to apply the knowledge gained to biomaterial designs, drug/gene delivery, and cell therapy. The Discher Lab pioneered studies of matrix elasticity directed stem cell differentiation, clarifying the effects on cells of a tissue stiffness scale illustrated as: brain < fat < muscle < bone. His lab was the first to measure and model nuclear mechanics in relation to mechanosensing, developing ‘omics methods and analytics to uncover scaling relationships between matrix and nuclear factors, including chromosome variations. His lab discovered the ‘phagocytic synapse’ between a macrophage and a cell displaying on its membrane or not a ‘marker of self’ protein that – with controlled inhibition – allows macrophages to be targeted to attack diseased tissue.
Keywords: stem cell differentiation; matrix stiffness and elasticity; nuclear plasticity; polymer synthesis and assembly; bioengineered therapeutics; cell engineering; mechanobiology
Working Group(s): Working Group 2: How do cells adapt to and change their mechanical environment; Working Group 3: How do cells remember their mechanical environment
Ram Dixit
Ram Dixit is a Professor of Biology at Washington University in St. Louis and Associate Director of Education for the Center for Engineering MechanoBiology.
Dixit’s research seeks to understand the interplay between biochemical and mechanical stimuli that control the growth and morphology of plant cells. In plants, the noncentrosomal cortical microtubule cytoskeleton determines cell shape by spatially organizing the rigid cell wall which specifies the direction of turgor-driven cell expansion. Anisotropic expansion in turn feeds back to alter cortical microtubule organization. The Dixit lab wants to elucidate the molecular mechanisms that generate and dynamically modify the organization of the cortical microtubules during plant growth and development. We are also interested in understanding how the cortical microtubule array guides the ordered deposition of cell wall constituents to affect the structure and mechanics of the cell wall. The lab uses a multi-disciplinary and multi-scale approach combining live-imaging, in vitro reconstitution at the single-molecule level, molecular genetics and computational modeling.
Keywords: plant cell morphogenesis; cortical microtubule cytoskeleton; plant cell wall; mechanobiology; microtubule plus-end and minus-end targeting proteins; microtubule severing; microtubule bundling; microtubule self-organization; kinesins
Working Group(s): Working Group 1: How do cells sense their mechanical environment?; Working Group 2: How do cells adapt to and change their mechanical environment?; Working Group 3: How do cells remember their mechanical environment?; Working Group 4: Crosscutting and Emerging Technologies
Marcus Foston
Marcus Foston, Ph.D., is Assistant Professor of Energy, Environmental & Chemical Engineering and Principal Investigator of the Bioproducts Engineering Lab at Washington University in St. Louis.
The Foston research program seeks to develop innovative and novel routes to exploit and utilize lignocellulosic biomass, taking advantage of materials involved in industries such as agriculture, papermaking, and forestry products. His primary research themes include sustainable conversion of biomass into chemicals using liquid-phase, heterogeneous catalysis; interfacing the catalytic depolymerization of biomass with microbial utilization; and understanding how plant cells respond to mechanical stimulus.
Keywords: conversion of biomass into chemicals; interfacing the catalytic depolymerization of biomass with microbial utilization; cell stimulus response; liquid-phase; heterogeneous catalysis; mechanobiology
Working Group(s): Working Group 2: How do cells adapt to and change their mechanical environment?; Working Group 3: How do cells remember their mechanical environment?; Working Group 4: Crosscutting and Emerging Technologies
Guy Genin
Guy Genin, Ph.D., is the Harold and Kathleen Faught Professor of Mechanical Engineering in the Department of Mechanical Engineering and Materials Science and a Professor of Neurological Surgery at Washington University in St. Louis. Genin is Chief Engineer for the Center for Innovation in Neuroscience and Technology. He also serves as the Chinese Ministry of Education Yangtze River Professor and McDonnell International Scholars Academy Ambassador to Xi’an Jiaotong University.
Genin is Co-Director of the Center for Engineering MechanoBiology, and the Primary Investigator of the WashU CEMB.
Genin’s research focuses on interfaces and adhesion in nature, physiology, and engineering. His current research focuses on interfaces between tissues at the attachment of tendon to bone, between cells in cardiac fibrosis, and between protein structures at the periphery of plant and animal cells.
Keywords: biophysical methods; biophysics; cell biology; extracellular matrix; substrates; periplasm configuration; cell adhesion and spreading; auxin; arabinogalactins; electrical signalling; mechanobiology
Working Group(s): Working Group 2: How do cells adapt to and change their mechanical environment?; Working Group 3: How do cells remember their mechanical environment?; Working Group 4: Crosscutting and Emerging Technologies
Yale Goldman
Yale E. Goldman, M.D., Ph.D., is Professor of Physiology at the University of Pennsylvania. He is also faculty in the Biochemistry & Molecular Biophysics and Cell & Molecular Biology graduate groups.
Dr. Goldman’s research seeks to relate structural changes to enzymatic reactions and mechanical steps of the energy transduction mechanism by mapping the real-time domain motions of the motor proteins and ribosomal elongation factors.
Motor proteins and GTP-binding proteins (G-proteins) share many structural and functional attributes. Molecular motors myosin, dynein and kinesin are prototype biological energy transducers that can be understood at a particularly fine level of detail. The obvious functional output (force and motion) allow the reaction sequence to be probed by single molecule biophysical, chemical and structural studies. A cyclic interaction between actin and myosin transforms free energy of splitting ATP into motion and mechanical work. Modified forms of this mechanism power other cell biological motions such as targeted vesicle transport and cell division. The Goldman research group is using novel biophysical techniques, including nanometer tracking of single fluorescent molecules, bifunctional fluorescent probes and infrared optical traps (laser tweezers) to map the real-time domain motions of the motor proteins.
Keywords: mapping domain motions of motor proteins and ribosomal elongation factors; mechanics of the energy transduction mechanism; enzymatic reactions; myosin; dynein; kinesin; nanometer tracking of single fluorescent molecules and fluorescent probes; optical traps; mechanobiology
Working Group(s): Working Group 1: How do cells sense their mechanical environment?; Working Group 3: How do cells remember their mechanical environment?; Working Group 4: Crosscutting and Emerging Technologies
Ajay Gopinathan
Ajay Gopinathan is Professor and Chair of the Department of Physics at the University of California, Merced (UCM). He is also a Director of the NIH-funded G-RISE (T32) graduate training program and of the NSF-funded CREST Center for Cellular and Biomolecular Machines at UCM. Our group uses theoretical and computational methods to explore the basic physics underlying a variety of biological transport processes ranging in scale from molecular motor transport to the collective motility of cells and organisms. We use theoretical concepts and methods from statistical mechanics, polymer physics, and elasticity as well as simulation techniques including molecular dynamics, coarse-grained Brownian dynamics, Monte Carlo simulations, and agent-based models. Examples of projects include understanding the role of mechanical coupling and interference among molecular motors in intracellular cargo transport, the role of cytoskeletal architecture and dynamics in cellular scale transport, and the effects of cell-cell interactions and heterogeneity in collectively migrating tumor clusters and developing vascular networks.
Su Chin Heo
Dongeun Huh
Dongeun Dan Huh, Ph.D., is the Wilf Family Term Chair and Assistant Professor of Bioengineering at the University of Pennsylvania and Principal Investigator of BIOLines, the Biologically Inspired Engineering Systems Laboratory.
Huh’s laboratory aims to develop innovative bioengineering tools and technologies using biologically inspired design principles and micro/nanoengineering approaches to improve human health and promote environmental sustainability. Huh’s research focuses primarily on developing i) microengineered biomimetic models of human organs (organs-on-chips), ii) self-assembled tissue/organ scaffolds, iii) cell-based self-regulating “smart” biomedical devices, and iv) efficient biomimetic transport systems. Huh explores the use of these bioinspired engineering systems for a variety of biomedical, pharmaceutical, and environmental applications.
Keywords: bioengineered therapeutics; devices and drug delivery; cellular engineering; tissue engineering; organs-on-chips; mechanobiology
Working Group(s): Working Group 4: Crosscutting and Emerging Technologies
Rajan Jain
Rajan Jain, M.D., is Assistant Professor of Medicine at the University of Pennsylvania and holds a graduate group affiliation with the Cell and Molecular Biology group.
The Jain research lab is interested in how genome organization shapes organogenesis. Overall, their research seeks to understand how cell identity is established and maintained. Jain’s team combines traditional molecular biology and genetic tools with innovative imaging and next-generation sequencing approaches to define how an embryonic stem cell progressively restrict their potential to give rise to various differentiated cell types in the body. The Jain group routinely leverages classic models of stem cell biology to dissect the underpinnings of cell fate decisions. The lab has a focus on cardiac biology but routinely works outside it.
It has become clear that the genome is folded and organized in a stereotypical manner in three- dimensional space. A major interest of the laboratory is deciphering how the organization of the genome in 3D space regulates genes and cell fate decisions. We are defining how the nuclear lamina, a network of proteins on the inner side of the nuclear membrane, interacts with chromatin to drive gene regulation and shape cell fate choices. Projects in the laboratory are geared towards defining the rules that govern nuclear lamina-chromatin interactions. We are also studying how mutations in LMNA and related proteins impact nuclear architecture and translate into cardiac dysfunction in patients.
Keywords: nuclear architecture; gene regulation; chromatin; epigenetics; 3D genome organization; heart development; stem cell biology; mechanobiology
Working Group(s): Working Group 2: How do cells adapt to and change their mechanical environment?; Working Group 3: How do cells remember their mechanical environment?
Paul Janmey
Description of Research Expertise
Research Interests
Cell and tissue mechanics
Intermediate filaments
Fibrous networks
Phosphoinositide signaling
Key words: actin, PIP2, cytoskeleton, gel, vimentin, fibrin, gelsolin.
Description of Research
Our lab studies the physical properties of fibrous networks and relates these properties to the mechanical responses of cells and tissues. In one project, we produce soft materials, usually hydrogels, to which cell adhesion proteins are linked to study how the stiffness of surfaces alters cell structure, function, and growth. Endothelial cells, fibroblasts, neurons and astrocytes each show unique dependence on both elastic and viscous properties of the substrate, and we seek to understand how they sense and respond to this mechanical cue. In related work we measure the structure and mechanics of cytoskeletal polymers using a variety of imaging, scattering, and rheologic methods. Further studies examine how changes in cell membrane structure mediated by inositol phospholipids lead to production of signals that remodel the cytoskeleton.
Rotation Projects
- Image morphology and cytoskeletal changes in actin or vimentin expressing fibroblasts, endothelial cells, and other cell types grown of soft materials.
- Fluorescence and atomic force microscopy of purified cytoskeletal polymers and associated motor proteins.
- Physical properties of fibrous networks, including those formed by cytoskeletal and extracellular matrix proteins
Lab personnel:
Robert Bucki
Visiting Professor
Medical University of Bialystok
Fitzroy (Jeff) Byfield
Research Specialist
Behnaz Eftekhari
Ph.D. Student, Bioengineering
Daniel Iwamoto
Post-doc
Katherine Kerr
Undergrad, REU and Purdue Univ.
Emile Kraus
Ph.D. student, Physics
J. Yasha Kresh
Visiting Professor
Drexel Univ. College of Medicine
Kalpana Mandal
Post-doc
Serena Omo-Lamai
Ph.D. student, Bioengineering
Dawei Song
Post-doc
Selected Recent Publications
Patteson AE, Vahabikashi A, Goldman RD, Janmey PA. 2020. Mechanical and Non- Mechanical Functions of Filamentous and Non-Filamentous Vimentin. Bioessays: e2000078.
Chaudhuri O, Cooper-White J, Janmey PA, Mooney DJ, Shenoy VB. 2020. Effects of extracellular matrix viscoelasticity on cellular behaviour. Nature 584: 535-46.
Shivers JL, Feng J, van Oosten ASG, Levine H, Janmey PA, MacKintosh FC. 2020. Compression stiffening of fibrous networks with stiff inclusions. Proc Natl Acad Sci U S A 117: 21037-44.
Charrier EE, Pogoda K, Li R, Park CY, Fredberg JJ, Janmey PA. 2020. A novel method to make viscoelastic polyacrylamide gels for cell culture and traction force microscopy. APL Bioeng 4: 036104.
Fatunmbi O, Bradley RP, Kandy SK, Bucki R, Janmey PA, Radhakrishnan R. 2020. A multiscale biophysical model for the recruitment of actin nucleating proteins at the membrane interface. Soft Matter 16: 4941-54.
van Oosten ASG, Chen X, Chin L, Cruz K, Patteson AE, Pogoda K, Shenoy VB, Janmey PA. 2019. Emergence of tissue-like mechanics from fibrous networks confined by close- packed cells. Nature 573: 96-101.
Engstrom TA, Pogoda K, Cruz K, Janmey PA, Schwarz JM. 2019. Compression stiffening in biological tissues: On the possibility of classic elasticity origins. Phys Rev E 99: 052413.
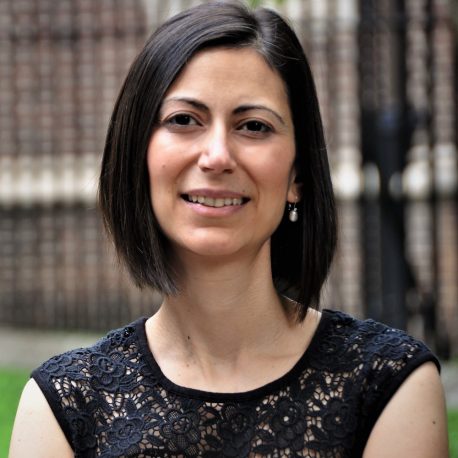
Melike Lakadamyali
One overarching goal of the Lakadamyali lab is to determine the interplay between epigenetics, the physical architecture of chromosomes and gene regulation. The human genome folds in a highly complex manner inside the nucleus and this folding is fundamentally important for regulating gene expression. However, how the genome is folded at the kilo-to-megabase genomic scales (10-300 nm spatial scale), which are the scales most relevant for gene regulation, has been difficult to determine since these length scales are inaccessible to conventional light microscopy. We have been overcoming this technical challenge by applying quantitative super-resolution microscopy methods to study the 3D organization of the genome in intact nuclei at the single cell level with nanoscale spatial resolution. We are determining how mechanical and chemical cues remodel the genome structure and how this remodeling regulates gene activity and cell fate determination.
Robert Mauck
Robert Mauck, Ph.D., is the Mary Black Ralston Professor for Education and Research in Orthopaedic Surgery and Professor of Bioengineering at the University of Pennsylvania. He is the Director of the McKay Orthopaedic Research Laboratory and the Biomechanics Core of the Penn Center for Musculoskeletal Disorders. Dr. Mauck co-directs the Program in Musculoskeletal Regeneration in the Penn Institute for Regenerative Medicine. Mauck is a Research Health Scientist at the Philadelphia VA Medical Center, and co-Director of the Translational Musculoskeletal Research Center (TMRC) at the VA.
Mauck also serves Co-Leader of Working Group 3 for the Center for Engineering MechanoBiology.
The Mauck Lab research program in soft tissue mechanobiology and tissue engineering aims to understand the physiologic and patho-physiologic processes that regulate the formation, maturation, and adult function of soft connective tissues. His group focuses on the engineering of musculoskeletal tissues, with a particular interest in restoring articular cartilage, the knee meniscus, and the intervertebral disc. Dr. Mauck’s team uses rigorous mechanical and molecular analyses to characterize native tissue structure and function and employs this information to enhance the functional properties of engineered constructs through focused technology development. Specifically, this work employs adult mesenchymal stem cells, custom mechanobiologic culture conditions, and novel cell scaffolding technologies.
Keywords: soft tissue mechanobiology; physiologic processes; patho-physiologic processes; tissue engineering; technological development; mesenchymal stem cells; mechanobiologic cultures; cell scaffolding; mechanobiology
Working Group(s): Working Group 2: How do cells adapt to and change their mechanical environment?; Working Group 3: How do cells remember their mechanical environment?; Working Group 4: Crosscutting and Emerging Technologies
Kara McCloskey, PhD
Kara E. McCloskey, PhD, is a founding professor in the Materials Science and Engineering Department at the University of California, Merced – a Hispanic serving institution. She is known for her work in directing endothelial cell (EC) fate from both human and mouse ESCs and induced pluripotent stem (iPS) cells, including a pioneering publication to identify and characterize the derivation of stable angiogenic and antiangiogenic ECs from stem cells. She has authored or co-authored approximately 50 peer-reviewed journal articles in areas from magnetic cell separation, stem cell differentiation, and tissue assembly. She is currently focusing her efforts examining cell-material interactions towards developing functional vascular and cardiovascular tissues.
Philip C. Nelson, PhD
Dr. Nelson is a professor of Biophysics in the Physics and Astronomy Department at UPenn.
Learn more about his work here.
Elijah Nyairo
Elijah Nyairo is an instructor of Chemistry and faculty member in the Department of Physical Sciences at Alabama State University. Nyairo is also a key member of the ASU Bone Tissue Regeneration Research Group.
Nyairo’s research interest is in nanostructured biomaterials for bone tissue repair and regeneration. His team aims to develop bioactive polymeric scaffolds from biodegradable polymers that mimic the functionality of native extracellular matrix (ECM). Scaffolds serve as temporary matrices that accommodate cells and support tissue regeneration. Scaffolds based on FDA approved biodegradable polymers and modified carbon nanofibers are fabricated using electrospinning to achieve improved mechanical properties, uncompromised cell growth and facilitate repair of damaged tissue.
Nyairo’s research group further explores the development of nanobiomaterials for drug delivery. One study is aimed at understanding the mechanism involved in the adsorption and release of therapeutics in a controlled manner from nanofiber polymer scaffolds. Scaffolds are fabricated by electrospinning polyvinyl alcohol (PVA) encapsulated with varying concentrations of detonated nanodiamonds (DND). The surface energy of the DND’s makes it relatively straightforward to adsorb or covalently attach therapeutic molecules. Thus, the team’s research focuses on the potential use of DND to more effectively deliver therapeutics and to minimize compatibility concerns associated with using carbon-based nanomaterials (i.e., cytotoxicity).
Keywords: polymeric scaffolds; nanobiomaterials; nanofibers; electrospinning; bone and tissue repair; biodegradable polymers; drug delivery; mechanobiology
Working Group(s): Working Group 2: How do cells adapt to and change their mechanical environment?
Michael Ostap
Michael Ostap, Ph.D., is Professor of Physiology and Principal Investigator of the Pennsylvania Muscle Institute. He is a Co-Leader of the CEMB Working Group 1.
The goal of the Ostap research team is to understand the cellular machinery responsible for powering cell movements and shaping the architecture of cells, tissues, and organs. The lab’s discovery-based research focuses on the role of the cytoskeleton, molecular motors, and signaling pathways in powering cell migration, muscle contraction, and the transport of internal cell compartments. The pathways investigated in the Ostap laboratory are crucial for several normal and pathological processes, including: cell and tissue development, endocytosis, wound healing, immune response, cardiomyopathies, and metastases of tumors.
Most of the team’s current efforts are focused on investigating cytoskeletal motors (myosin, dynein, and kinesin). These nano-machines use chemical energy stored in cells (in the form of ATP) to generate mechanical force and motion. Cytoskeletal motors are the engines that power muscle contraction, cell migration, intracellular transport, cell division, and cell shape. Ostap’s team is determining how these motors work at the molecular level, how they are physically connected to the machinery they are powering, how they are regulated, how they interact with other motors and signaling networks, and how their fundamental biophysical parameters impact cell function. The lab uses a range of biochemical, cell biological, single-molecule, and other biophysical techniques to better understand these proteins in health and disease.
Keywords: cytoskeleton; molecular motors; cell signalling; muscle contraction; cell structure; intracellular transport; myosin; dynein; kinesin; mechanobiology
Working Group(s): Working Group 1: How do cells sense their mechanical environment?
Chinedum Osuji, PhD
Dr. Osuji is an Eduardo D. Glandt Presidential Professor
Chemical and Biomolecular Engineering (CBE) at University of Pennsylvania. Learn more about his research here.
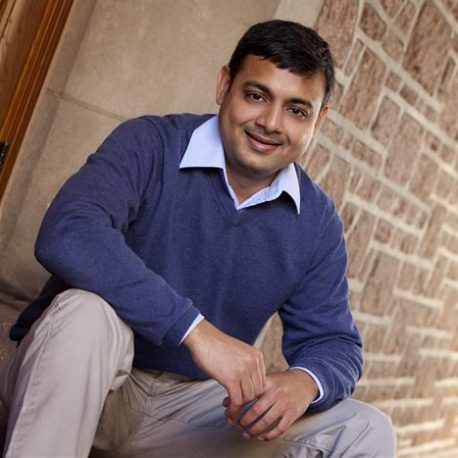
Amit Pathak
2018 SEED project summary: Collectively migrating cells sense matrix stiffness through adhesions and cytoskeletal forces. We have recently shown that mechanical priming of epithelial cells on a stiff ECM enhances their future migration, even after they move to a soft ECM. This storage of past mechano-activation in migratory cells depends on nuclear YAP localization. In this project, we investigate biophysical mechanisms that connect nuclear YAP to cellular forces and collective cell migration. Based on experimental findings, we are also building a computational framework for collective cell migration across matrices of dissimilar stiffness through rate-dependent mechanotransduction. Through this integrated approach, we aim to understand how mechanical priming and memory might be regulated by the crosstalk of nucleus shape, YAP localization, cytoskeletal machinery, and cell migration, all operating at multiple time and length scales.
Benjamin Prosser
Dr. Prosser received his B.S. in Biomechanics from Wake Forest University in 2005. He then earned his Ph.D. in Molecular Medicine from the University of Maryland School of Medicine in 2009, where he studied muscle electrophysiology in the lab of Martin Schneider. Dr. Prosser completed his post-doctoral fellowship in Molecular Cardiology with Jon Lederer at the University of Maryland Biotechnology Institute in 2013, where he was awarded the Outstanding Post-Doctoral Scholar Award for his work on cardiomyocyte mechanosignaling. Dr. Prosser started his own group at the University of Pennsylvania Perelman School of Medicine in 2014, where he is now an Assistant Professor of Physiology and the Associate Director of the historic Pennsylvania Muscle Institute. The Prosser lab focuses on the mechanobiology of the heart – the mechanisms that regulate the ability of the heart cell to generate force, and how external forces in turn feedback to influence myocyte physiology and pathology. In recognition of the lab’s early work, Dr. Prosser was named the Outstanding Early Career Investigator by the American Heart Association and an Outstanding Young Investigator of the Penn School of Medicine in 2017. Starting in January of 2021, Dr. Prosser will serve as Lead Coordinator of a Leducq Transatlantic Network of Excellence, an international research consortium focused on the role of the cytoskeleton in cardiac health and disease.
Vivek Shenoy
Vivek Shenoy, Ph.D., is the Eduardo D. Glandt President’s Distinguished Professor in the School of Engineering and Applied Science with appointments in the Departments of Materials Science and Engineering, Mechanical Engineering and Applied Mechanics, and Bioengineering. He is Director of the Center for Engineering MechanoBiology.
Professor Shenoy’s research team focuses on developing theoretical concepts and numerical methods to understand the basic principles that control the behavior of both engineering and biological systems. A significant challenge in modeling the engineering and biological systems examined by Shenoy’s team is that important processes involve the coupling of both small-scale (atomic or single molecule) phenomena and long-range (elastic, electromagnetic) interactions over length scales of hundreds of nanometers. His group addresses these issues by combining atomic scale simulation methods with continuum or mesoscale theories and by adapting insights from condensed matter physics, solid mechanics, chemistry, materials science and applied mathematics.
Keywords: Cell-matrix interactions, Chemo-mechanical models for cell adhesions and motility, Nuclear mechanotransduction, Mechanics of fibrous networks
Working Group(s): Working Group 2: How do cells adapt to and change their mechanical environment?; Working Group 3: How do cells remember their mechanical environment?; Working Group 4: Crosscutting and Emerging Technologies
Tatyana Svitkina, PhD
Tatyana Svitkina, Ph.D., is a Professor at the Department of Biology in the School of Arts and Sciences.
Dr. Svitkina’s team investigates roles of the actin cytoskeleton in various cellular activities, such as cell motility, morphogenesis, cell shape determination, cell-cell interactions and membrane organelle dynamics. Their distinctive approach toward understanding cytoskeleton functions is to take advantage of high resolution structure of the cytoskeleton using platinum replica electron microscopy (PREM) and link it to cellular activities and roles of individual proteins. Their studies have produced many critical insights into functions of various cytoskeletal systems and individual proteins in a range of generic and specialized cell types.
Keywords: cytoskeleton, actin, myosin, microtubules, electron microscopy, cell motility, cell adhesion, neuronal morphogenesis
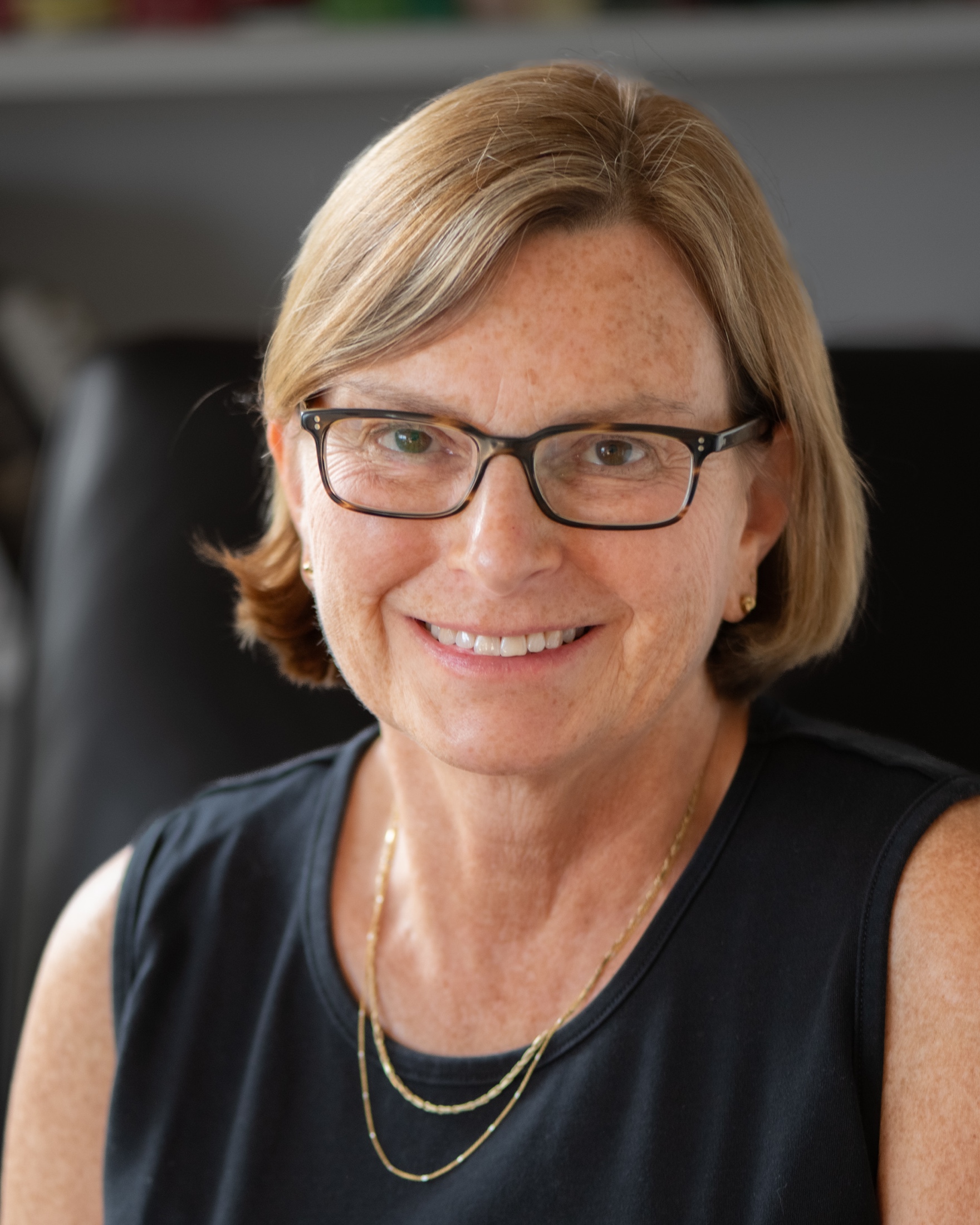
Rebecca Wells
Rebecca Wells, M.D., is Professor of Medicine, Bioengineering, and Pathology and Laboratory Medicine with graduate area affiliations in Bioengineering, Cell and Molecular Biology, and Pharmacology at the University of Pennsylvania. She is the Director of Education of the Center for Engineering MechanoBiology.
The Wells Lab focuses on the mechanism and consequences of hepatic fibrosis. Overall, the goal of the research team is to develop a unified and comprehensive model of liver fibrosis that incorporates multiple cell types, soluble and secreted factors, matrix proteins, and local and regional mechanical factors.
Liver fibrosis results from the deposition of excess, abnormal extracellular matrix by myofibroblasts derived from non-fibrogenic cells that undergo “activation” in the context of chronic liver injury. Fibrosis in the bile duct is a similar matrix-driven process, although the identity of the myofibroblast populations and the chronic vs. acute nature of the injury are not known.
The Wells Lab is investigating the mechanisms of fibrosis in four ways: a) by studying the matrix, mechanical, and soluble factors that influence fibrosis, including the activation of myofibroblast precursor populations; b) by studying the interactions between proteoglycans and collagen as it relates to fibrotic diseases; c) by identifying new fibrogenic cell populations and new means of studying previously identified cells; and d) by applying the results of experiments with isolated cells to whole animal models and to the study of human diseases, including hepatocellular carcinoma, fatty liver disease, and biliary fibrosis.
Keywords: Hepatic stellate cells, liver fibrosis, TGF-ß, portal fibroblasts, biliary atresia, liver mechanics, fibronectin; mechanobiology
Working Group(s): Working Group 2: How do cells adapt to and change their mechanical environment?; Working Group 3: How do cells remember their mechanical environment?; Working Group 4: Crosscutting and Emerging Technologies
Faculty Alumni
Richard Assoian
Dr. Richard Assoian is Professor of Pharmacology and a member of the Center for Cancer Pharmacology at the University of Pennsylvania. Assoian was a Co-Director of the Center for Engineering MechanoBiology from 2017-2021.
Assoian’s research lab explores how cells sense changes in the physical properties of their microenvironment and how they convert this information into chemical signals, behavior and function. Within this broad area, his team seeks to understand how physiological and pathological changes in the stiffness of the extracellular matrix (ECM) affects adhesion receptor signaling, the actin cytoskeleton, and fate decisions such as proliferation, migration and differentiation. Assoian’s group performs mechanistic analyses in cell culture, use genome- and proteome-wide approaches, assess mechanical properties of tissues and cells, and ultimately test physiological and pathological relevance in mouse models of vascular aging, injury, and atherosclerosis.
Keywords: extracellular matrix; microenvironment; adhesion receptor signaling; mechanotransduction; integrins; cadherins; actin; cytoskeleton; focal adhesions; matrix remodeling; deformable substrata; micropatterning; cell cycle; proliferation; cyclin-dependent kinases; mouse modeling; tissue mechanics; cardiovascular biology; progeria; mechanobiology
Working Group(s): Working Group 2: How do cells adapt to and change their mechanical environment?; Working Group 3: How do cells remember their mechanical environment?
Elizabeth Haswell
Elizabeth Haswell earned her PhD in Biochemistry at the University of California, San Francisco in 2000, working on chromatin remodeling in yeast. She then switched to plant systems for her postdoctoral work as a Life Sciences Research Fellow at Caltech, where she fell in love with plant mechanobiology. In 2007, she joined the Biology faculty at Washington University in Saint Louis, where she is now a Professor and HHMI-Simons Faculty Scholar. Her research group aims to identify the molecular and cellular mechanisms by which plants perceive force, with a particular focus on mechanosensitive ion channels. She teaches a course on Plant Biology and Genetic Engineering. Liz is a Senior Editor for The Plant Cell and a Deputy Editor at Science Advances. She serves on the Multinational Arabidopsis Steering Committee and is an AAAS Council Delegate. She is an advocate for science communication and for an academic culture that values sustainability, diversity, and authenticity. She is also a co-host of The Taproot, a Plantae podcast.
Affiliate Members
Yongho Bae
Dr. Yongho Bae is an Assistant Professor of Pathology and Anatomical Sciences at the University at Buffalo. He obtained his M.S. in Biochemical Engineering from Rutgers University and Ph.D. in Bioengineering from the University of Pittsburgh. During his Ph.D. studies, he explored the impact of profilin-1 on breast cancer cell motility. Afterwards, he completed a post-doctoral fellowship in Pharmacology at the University of Pennsylvania, where he focused on investigating the effects of ECM stiffness on cell cycle progression.
Dr. Bae’s lab is focused on understanding the impact of changes in ECM stiffness on vascular smooth muscle cell behavior. His research has demonstrated that a stiff ECM can lead to pathological cell behaviors, such as increased migration and proliferation, in microenvironments associated with diseases such as vascular and cardiovascular disease. Through this research, his lab aims to gain a deeper understanding of the underlying mechanisms behind these pathological behaviors and to develop targeted therapies for these diseases.
Key words: Mechanobiology, Mechanomedicine, Vascular Biology, Atomic Force Microscopy, Stiffness, ECM, Survivin, Lamellipodin
Alexander Buffone, Jr.
Alexander Buffone is an Assistant Professor in the Departments of Biomedical Engineering in the Newark College of Engineering at the New Jersey Institute of Technology (NJIT). He is also the Director of the Cellular Motion Laboratory.
The research in the Buffone Cellular Motion Lab lies at the intersection of Glycobiology, Cell Migration, Mechanobiology, and Engineering. Our lab utilizes a combination of CRISPR genetic engineering, microfludics, and models of acute inflammation, to study how both cancer and immune cells interact and move in their environment. By disrupting the cell surface, mechanosensitive receptors, and glycocalyx composition, our ultimate goal is to fine-tune and precisely control cell migration for therapeutic benefit during disease states.
Keywords: Immune cell, cancer, cellular motion, migration, glycobiology, mechanobiology, microfluidics, genetic engineering.
Xuemei Cheng
Xuemei (May) Cheng, Ph.D. is Associate Professor and Department Chair of Physics at Bryn Mawr College. She is Director of the Bryn Mawr Nanomaterials and Spintronics Lab.
May’s research group focuses on the fabrication, characterization and application of nanoscaled materials. Current projects include: templated electrochemical deposition of nanoscaled materials for energy and medical applications; time-resolved imaging of spin dynamics in magnetic nanostructures; and x-ray magnetic circular dichroism study of multiferroic materials.
Keywords: nanostructured materials; spintronics; fabrication and modeling of nanostructured materials; time-resolved imaging of spin dynamics; electrochemical deposition; x-ray magnetic circular dichroism studies; nanoscience; mechanobiology
Working Group(s): Working Group 2: How do cells adapt to and change their mechanical environment?
Elise A. Corbin, PhD
Elise A. Corbin, PhD, is an Assistant Professor in Biomedical Engineering and Materials Science and Engineering at University of Delaware.
Our lab focuses on the investigation of the complex interaction of cells and tissues with their environment through the use of a new generation of dynamic materials and devices. Interactions between physical forces, rheological properties, and biochemical cues regulate virtually all aspects of cell physiology. Therefore, mimicking essential features of physiological and pathological conditions is essential for elucidating fundamental cell and tissue adaptations and translating basic research to the clinic. From this perspective, technologies capable of precisely varying the timing, magnitude, and combinations of biomechanical and biochemical inputs provide great investigative power.
Research Keywords: Dynamic microenvironments, biomaterials, mechanical stiffness, spatiotemporal dynamics
Elliot L. Elson, PhD
University of Washington in St. Louis
Professor, Emeritus, Biochemistry and Molecular Biophysics
https://biochem.wustl.edu/elelab/
Peter A. Galie
Peter A. Galie, Ph.D., is an Associate Professor of Biomedical Engineering at Rowan University in Glassboro, New Jersey. Galie’s laboratory studies the effects of fluid and solid mechanics on in vitro models of blood vessels in the central nervous system. Their work incorporates computational and experimental fluid dynamics to characterize the shear stress applied by blood flow, as well as magnetorheology to rapidly and reversibly tune the mechanical properties of cell-seeded hydrogels. The laboratory aims to use this research to identify treatments for neurovascular disease and to fabricate vascularized scaffolds to regenerate the injured spinal cord.
Keywords: fluid shear stress, cell-matrix interactions, blood-brain barrier, magnetorheology, 3D-printing, transcriptomics
Murat Guvendiren
2018 SEED project summary: Stem cells reside in a complex and dynamic microenvironment, where they sense geometrical, chemical and mechanical cues, and respond to it. There is increasing evidence that curvature of the external environment, including at the scale of a cell length, directly affects stem cell behavior. Yet, existing cell culture systems fail to accurately mimic the architecture experienced by stem cells in 3D. This proposal will address this gap. The main goals of this proposal are: (i) to develop a fundamental understanding of the effects of “cell-scale” (on the order of a cell length) curvature on cell function, and (ii) to determine the effect of matrix stiffness and ligand density on the ability of a cell to sense and respond to curvature. We propose to develop 3D bioprinted hydrogel devices from novel bioink formulations that will enable unparalleled control over curvature, stiffness, and ligand expression.
Nathaniel Huebsch, PhD
University of Washington in St. Louis
Assistant Professor, Biomedical Engineering
https://huebschlab.wustl.edu/home/
Deep Jariwala
2018 SEED project summary: This SEED aims to probe cell-matrix adhesion by use of atomically thin conducting materials such as graphene sheets as interfacial layers. By spatially patterning and varying the conductivity of graphene sheets, the interactions of adhesion molecules such as integrin. More specifically the atomically thin nature of graphene allows electric-field to penetrate through it thereby having a potential to affect local adhesion of integrin which contains calcium or magnesium ions by application of electric voltage. A proof of concept demonstration will open doors to electrical control of cell adhesion, growth, and proliferation.
Eleni Katifori, PhD
Dr. Katifori is an Associate Professor in the Department of Physics and Astronomy at the University of Pennsylvania. Learn more about her research here.
Minwook Kim, Ph.D.
Minwook Kim, Ph.D., is an Assistant Professor in Molecular Biology at Virtua Health College of Medicine & Life Sciences of Rowan University.
Our lab focuses on the investigation of synovial joint development and regeneration. We use the principles of developmental biology and bioengineering to understand how synovial joints form during embryonic joint morphogenesis and how joint tissues maintain their functions throughout adulthood. Then, we use this insight to develop engineered tissues that recapitulate native structures and functions of articular cartilage for clinical applications.
Research Keywords: Synovial joint development, Articular cartilage, Hyaluronic acid (HA), HA metabolism, Cell and Tissue engineering
J. Yasha Kresh, MB, PhD, FACC, FAHA, FAPS
My professional involvement in cardiovascular basic research and its clinical translation spans more than three decades. The research conducted has emphasized the unity of knowledge, linking (bio)medicine, (bio)physics, (bio)mathematics and (bio)engineering. The ongoing basic research encompasses integrative aspects of cardiovascular ‘form-function’, studying the structural and functional outcomes of intercellular and extracellular mechanical communication. The applied research targets therapies to repair / replace the failing pump function of the heart using regenerative tissue engineering and novel mechanical cardiac replacement device design strategies. The broad ranges of research projects (e.g., cardiac biomechanics, electrophysiology, myocardial energetic, regeneration) that have been pursued reflect this highly interdisciplinary approach.
More recently, attention has been directed to investigations in the area of cellular mechanobiology and conditions that lead to cardiac muscle structural and functional remodeling (single and collective cell behavior). In particular, exploring the key modulators involved in cardiac myocyte / fibroblast cytoskeletal plasticity and epigenetic reprogramming in response to mechanical cues of the local microenvironment; whether transmitted through the extracellular matrix (integrin-ECM interactions) adhesion / tethering and/or cell-cell adherens– mediated tugging forces.
Presently, we have two ongoing projects designed to elucidate the spatiotemporal relationship between 3-D helical cardiac myofiber structure, torsional motion and vortical entrainment of blood flow. The observation that spiral flow is highly efficient form of fluid propulsion, motivated its emulation (translation) in the design of a new generation mechanical cardiac-assist modalities and indwelling cavopumonary blood pumps for the failed single-ventricle (Fontan) circulation.
Wesley R. Legant, PhD
University of North Carolina
Assistant Professor, Pharmacology
Assistant Professor, Biomedical Engineering
http://legantlab.org/
Kenneth B. Margulies, MD
Hospital of University of Pennsylvania
Professor, Physiology
https://www.pennmedicine.org/providers/profile/kenneth-margulies
Gretchen A. Meyer, PhD
Dr. Meyer is a professor in the Department of Physical Therapy and lead the Integrative Muscle Physiology lab, which focuses on understanding how changes at the cellular level in skeletal muscle affect muscle structure and function, and, in turn, how pathological changes in muscle affect cellular processes. Our main projects are focused on the interaction between muscle and fat. Though much less studied than its “pathological sibling”, fibrosis, the formation of fat in and around muscle is equally prevalent across myopathies. Our research investigates fat’s effect on muscle function and how that effect is regulated at cellular and tissue levels.
Sharon Celeste Morley
2018 SEED project summary: Specialized immune cells in the lung, called alveolar macrophages, control how much inflammation the lungs generate after inhalation of an irritant (like smoke) or a germ. Deciding how much inflammation to cause is important. If the lungs don’t generate enough inflammation, the body won’t be able to clear the irritant or germ. If the lungs generate too much inflammation, the healthy tissue can be damaged. Our laboratory studies a protein in the alveolar macrophage, called L-plastin, which helps the macrophages stick to the lung tissue. We propose that one-way alveolar macrophages decide how much inflammation to cause is by sensing lung stiffness—healthy lungs are very soft, but diseased lungs get very stiff. We also propose that L-plastin is a protein that helps the macrophages sense lung stiffness. Our CEMB project investigates how L-plastin helps the alveolar macrophages sense stiffness and how sensing stiffness helps the macrophages decide how much inflammation to cause.
Guilherme Nader
Guilherme Nader, Ph.D. is an Assistant Professor in the Department of Pathology and Laboratory Medicine at the Children’s Hospital of Philadelphia and Univ. of Pennsylvania, Perelman School of Medicine. Guilherme Nader is originally from Sao Paulo-Brazil. He earned his Ph.D. in Molecular and Cellular Biology from Columbia University where he studied the trafficking of adhesion receptors during cell migration. Subsequently, Guilherme moved to Paris for his postdoctoral training at Institut Curie where he studied the consequences of nuclear deformation and rupture to cell function and fate. The Nader lab seeks to reconstitute the complex interface between mechanical and chemical signaling during cell migration and growth in dense microenvironments and tissue crowding. We employ microfluidics and microfabrication to apply controlled and precise confinement to cells and mechanical stress to the nuclei. We combine these tools with diverse fluorescence microscopy techniques to investigate how the tissue environment regulates cell function through its impact on nuclear deformation and integrity.
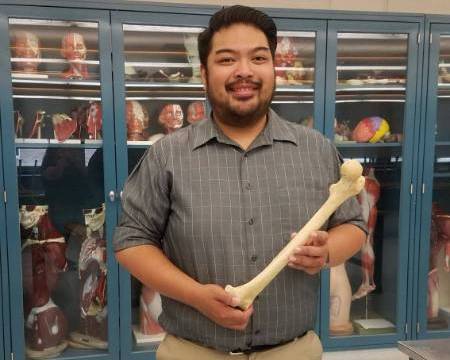
Kathleen J. Stebe, PhD
Professor, Chemical and Biomolecular Engineering
University of Pennsylvania
https://stebelab.seas.upenn.edu/
Kathleen J. Stebe, PhD
Professor, Chemical and Biomolecular Engineering
University of Pennsylvania
https://stebelab.seas.upenn.edu/
Lucia Strader
Lucia Strader, Ph.D.,is Associate Professor of Biology at Duke University.
Animals require cell movement to determine the final form of organs. Because there is no morphogenic cell movement in plants, and because the cell wall is usually formed immediately after cell division, plant morphogenesis depends upon careful control of both cell division and cell expansion. The auxin indole-3-acetic acid (IAA) is a critical plant hormone, controlling both cell division and cell expansion and thereby orchestrating many developmental events and environmental responses. Normal plant morphogenesis and environmental responses require modulation of auxin levels and responsiveness by interaction with other hormones, controlling biosynthesis, regulating transport, and managing storage forms. As such, auxin is critical for generating the mechanical forces necessary to drive plant growth and is responsible for growth in response to mechanical forces.
Current research in the Strader lab focuses on several projects using the model plant Arabidopsis thaliana:
- Regulation of AUXIN RESPONSE FACTORs
- Auxin regulation of cell mechanics
- The role of the MAP kinase phosphatase IBR5 on mediating responses to the plant hormones auxin, abscisic acid, and ethylene
- The role of IBA-derived auxin in plant development
Keywords: auxin, plant morphogenesis, cell division and expansion, environmental response, biosynthesis, cell transport, storage forms, indole-3-butyric acid (IBA), mechanobiology
Spencer Szczesny, PhD
Spencer Szczesny, Ph.D., is an Assistant Professor at the Pennsylvania State University with a joint appointment in the Department of Biomedical Engineering and the Department of Orthopaedics and Rehabilitation.
Dr. Szczesny leads the Tendon Multiscale Mechanics and Mechanobiology Lab, which examines how cells in tendon sense the mechanics of their local microenvironment (e.g., strains, stiffness, topography) and how their response drives changes in tissue mechanical properties during tendon degeneration, repair, and development. The ultimate goals of this work are to identify the causes of tendon pathology, discover novel therapeutic options, and direct the design of biomaterials that can recapitulate the behavior of native tissue. Furthermore, this research will produce fundamental knowledge regarding the feedback loop between local tissue mechanics and cellular mechanobiology through the use of multiscale mechanical testing, computational modeling, cell and tissue culture, and biomaterial fabrication.
Keywords: tendon, multiscale mechanics, mechanobiology, computational modeling
Kyle Vining, DDS, PhD
Dr. Vining holds a PhD in bioengineering from Harvard University (2020), a DDS from the University of Minnesota School of Dentistry (2014), and a BS in biomedical engineering from Northwestern University (2009). He was a postdoctoral scientist at the Dana-Farber Cancer Institute prior to joining Penn. He was the first faculty member recruited to be part of the Center for Innovation & Precision Dentistry (CiPD) at Penn Dental Medicine. A primary focus of Dr. Vining’s research has been targeting mechanical regulation of tissue inflammation in bone marrow disease and head and neck cancer. He is also developing strategies to target inflammation and promote tissue repair and regeneration in oral and craniofacial diseases. Among his projects in the area of biomaterials development, he engineered a novel antimicrobial coating for titanium dental implants and developed a new polymeric dental material that supports differentiation and proliferation of dental pulp stem cells for regenerative dentistry applications. His most recent work was published in Nature Materials.
Jessica Wagenseil, PhD
Professor, Department of Mechanical Engineering
Washington University of St. Louis
https://wagenseil.mems.wustl.edu/
John W. Weisel, PhD
Professor, Department of Cell and Developmental Biology
University of Pennsylvania
https://cdb.med.upenn.edu/people/john-weisel/
Shu Yang, PhD
Shu Yang is a Professor in the Departments of Materials Science & Engineering, and Chemical & Biomolecular Engineering in the School of Engineering and Applied Science at University of Pennsylvania (Penn). She is also Director of the Center for Analyzing Evolved Structures as Optimized Products (AESOP).
By combining chemistry, fabrication at the convergence of top-down and bottom-up approaches, and structure-property characterization together with insights from soft matter physics and soft mechanics and device fabrication and manipulation, the Yang lab aims to create novel materials and devices from nano- to meterscale. Special interests involve synthesis and engineering of well-defined polymers, gels, colloidal particles, biomaterials, and organic-inorganic hybrids with controlled size, shape, and morphology over multiple length scales, and understand chemo-mechano-opto behaviors and stimuli-responsiveness in soft and geometric substrates.
Keywords: materials synthesis, surface patterning, directed assembly, surface/interfacial behaviors, soft mechanics, environmental responses, geometric effect
Faculty from a diverse array of institutions and scientific backgrounds, all contributing to research in mechanobiology.
Staff support research, education, and diversity missions at the University of Pennsylvania and Washington University in St. Louis.
Postdoctoral fellows, graduate students, and undergraduates who have found success in many fields, including academia and industry.